Quantum physics is weird. Tiny quantum objects, like electrons and photons can behave like both waves and particles. They can tunnel through barriers that cannot be tunnelled through according to classical physics. They can be entangled together so that one part influences the other(s) instantly even if they are far apart. Measurements are based on probability, and outcomes are not predicted with certainty.
“For the first time, physicists will have tools that are naturally tailored to study problems that are impossible on classical computers. With these tools, physicists will be able to study quantum problems in condensed matter, cosmology, and more.”
Quantum computing involves harnessing the weirdness of quantum physics using quantum bits, or qubits, which are tiny two-level quantum systems. Information is encoded in qubits and computational operations can be performed that are impossible using classical bits. This potential has caused a surge in interest in quantum computing in the past few decades, and will likely accelerate as technology continues to advance.
One issue is that qubits are fragile and will experience the effects of noise when they interact with their external environment, and this introduces errors in computations. These errors have to be accounted for in order to obtain useful output. One way to mitigate, or lessen, these errors is by using algorithms from machine learning known as neural networks.1
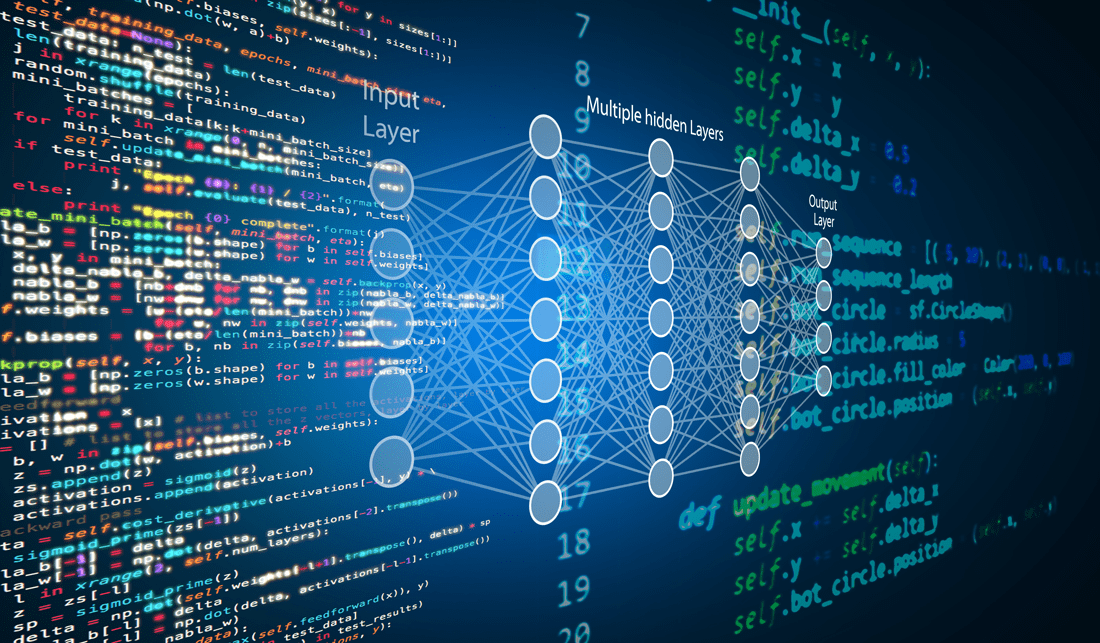
Figure 1: Artistic rendering of a neural network
This technique—called neural error mitigation—is actively being researched by Elizabeth Bennewitz, a quantum computing and machine learning researcher at 1QBit, together with Pooya Ronagh2 and fellow collaborators. “We have recently written a paper on our neural error mitigation method. Since defending my master’s thesis, I, along with my collaborators, have been applying neural error mitigation to more-complex models and systems,” she says.
The team is now focused on applying their quantum computation work to the fields of condensed matter physics and quantum chemistry.
Their work is timely for quantum technology. The number of qubits, and how deep they are connected in quantum circuits, are severely limited by noise. Boosting quantum computation with machine learning tools—like neural networks—will help bridge the gap between current classical and new quantum technologies.
Industry Broadens the Scope and Complements Academia
Elizabeth has found her research outside of academia to be dynamic. “I love the research environment and people at 1QBit,” she says. “I have enjoyed engaging with other team members from different technical backgrounds and hearing about their interesting work,” she continues. “I feel very appreciative to work in an industry where I can apply my physics background to my research.”
And, as words of advice to other students in physics, she wanted to emphasize that there is more than one path forward to having a career in physics. “In the past,” she says, “I felt that there was only one choice—to stay in academia. I’ve really valued my time at 1QBit not only because it offered another avenue to apply my physics background but also because of the opportunity to delve into the fascinating field of quantum computing.”
Elizabeth’s exposure to quantum computing research at 1QBit motivated her to pursue a PhD in physics. She notes, “I want to study how quantum computers can be used to solve problems in physics and to contribute to the design of better quantum technologies by working closely with experimentalists. I love the interdisciplinary nature of this field and am excited to learn and explore.”
Why is Quantum Computing Research Important?
Building a quantum computer is an exciting challenge that promises to transform computing. Unlike classical computers, quantum computers process information using the characteristics of quantum physics. Classical computers represent information using bits of information represented as 0 or 1.
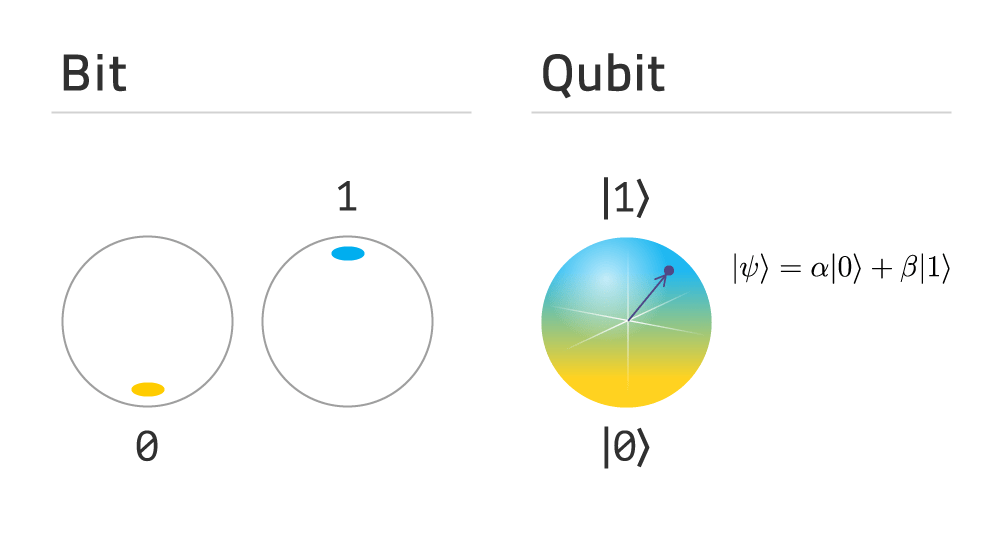
Figure 2: Comparing a bit to a qubit
Quantum computers represent information using qubits. Qubits can be in the states of 0, 1, or any combination of 0 or 1 because of a quantum effect known as quantum superposition. A given qubit can be visualized as existing at any point on the surface of the sphere in Figure 2.
More complex information can be represented using a single qubit than a single bit. And, the number of combinations increases exponentially with the number of qubits, allowing for more complex operations to be performed using quantum algorithms compared to classical algorithms. There is the potential for speed-ups for certain computations, and allows quantum computers to solve some problems that are impossible on a classical computer.
For a long time, it has been extremely difficult to use a classical computer to simulate—and therefore study—large systems that have many quantum particles. Unlike classical computers, quantum computers can naturally account for quantum effects. “For the first time, physicists will have tools that are naturally tailored to study problems that are impossible on classical computers. With these tools, physicists will be able to study quantum problems in condensed matter, cosmology, and more,” Elizabeth reflects.
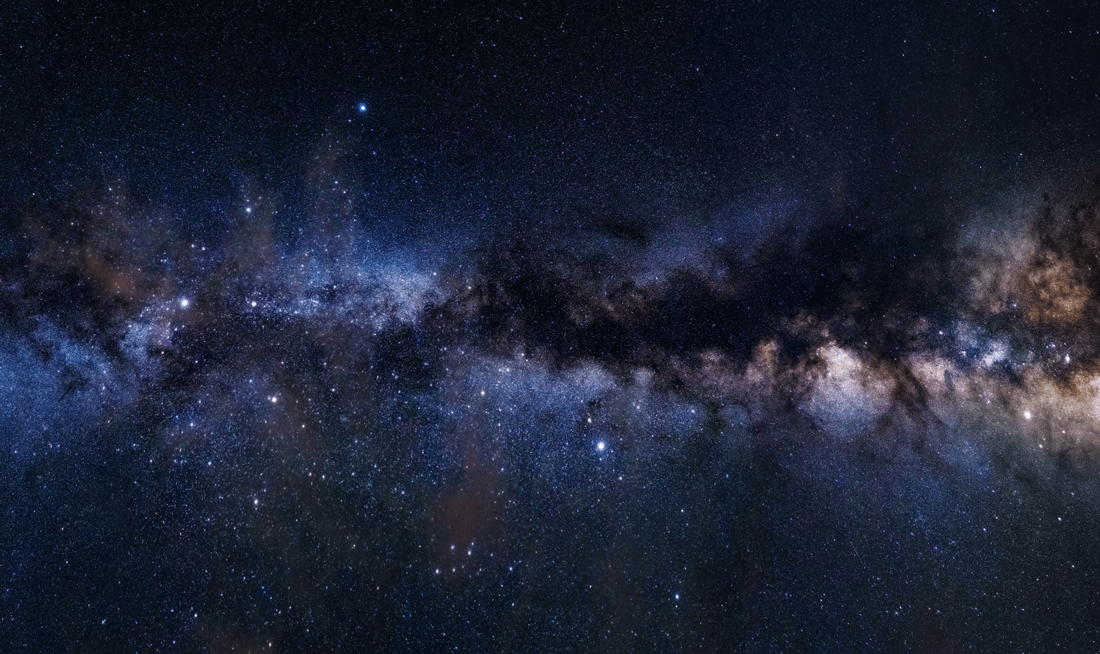
Figure 3: Panoramic picture of the Milky Way galaxy
Thanks in no small part to her research opportunities at 1QBit, Elizabeth had several strong options for pursuing her PhD on full scholarships. “I decided to pursue my PhD studies at the University of Maryland because of their expansive research in quantum information and quantum computing. I am thankful that I had a lot of great options which made my decision really hard,” she relates.
Elizabeth is looking forward to bringing her 1QBit experience to her studies at the University of Maryland. Researchers at the university have created a thriving research ecosystem for quantum information and quantum computing. “I am excited to be in a research environment with such a wide breadth of quantum computing researchers, from experimentalists to quantum information theorists to computer scientists to physicists,” she notes.
Elizabeth’s research on neural error mitigation is set to appear in the coming days, and will be accessible here. For a wide scope of topics in advanced and quantum computing and other research conducted at 1QBit, subscribe to our blog.
If you are interested in pursuing a career at the forefront of academia and industry, please visit our careers page.
Footnotes
1 More details can be found in the first and second parts of this three-part series.2 Pooya is a Research Assistant Professor at the Department of Physics & Astronomy and the Institute for Quantum Computing (IQC) at the University of Waterloo; Scientific Lead at the Perimeter Institute Quantum Intelligence Lab; and Director of the Hardware Innovation Lab of 1QBit.